Sorry for the delay, but this one has taken a lot of time and thought to put together (and reading some 80 odd Covid research papers). My goal in this edition of Covid Science Theater 2020 is to talk about what happens when the virus enters our body, infects our cells and subsequently leads to either mild disease or more severe infections. It’s going to be a fairly dense article, but I’ll do my best to keep the science and terminology to something generally understandable and hopefully educational.
For those who don’t want to delve too much into the specifics of all the virology, immunology and pathology I’ll provide a short 1 paragraph set of clifs notes here. The virus most commonly enters the host through mucus membranes (eyes, nose, mouth) and infects vascular endothelial cells and cells of the lungs, kidneys, GI tract and begins to replicate. The body initially responds to the virus through a host of Innate Immune mechanisms; these generic counter measures are deployed against all invading pathogens as a first line defense and are not specific to the invading pathogen. Unfortunately, these initial immune responses aren’t always adequate to contain the virus (the virus sometimes evades destruction, other times the virus just overpowers the immune response) so our body deploys a second type of response known as the Adaptive Immune response. In this phase, T-cells and B-cells are primed to respond to the specific infectious agent (here, SARS-CoV-2). Often this two-pronged approach works to contain the infection, eliminate the virus and build up lasting memory to subsequent infections. Unfortunately in some people the virus spreads too rapidly and the immune response doesn’t respond appropriately, leading to destruction of their organs (notably the lungs) and potentially death. Sometimes this more severe outcome is caused by the virus itself, but more often it seems to be caused by an overzealous immune system trying to play catch-up. So there’s the quick and dirty; in the following paragraphs I’ll go into more detail about Viral Entry/Binding/Replication, Early Cellular Responses, Clinical Symptoms, Adaptive Immune Response and What Happens and Why the Immune System Sometimes Fails.
The information in the following paragraphs comes from a combination of basic immunology principles (Kuby Immunology textbook), observations and early research released about Covid-19 and conclusions drawn from earlier studies of SARS-CoV-1 (a virus that is very similar to the current SARS-CoV-2, but with some caveats of course). As Covid-19 is still a new disease, we are constantly learning new things about the virus, infection cycle and pathology, so while what I outline here is based on a lot of research, there are definitely aspects of this virus that we don’t fully understand, and need further investigation.
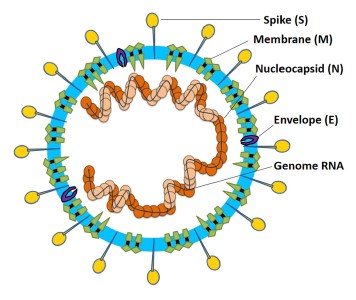
Viral Entry and Replication
Coronaviruses get their name from the hallmark shape, a circular capsid (or shell) that is spiked with proteins on the outside and sheltering the virus genetic sequence on the inside. The Spike (S), Membrane (M) and Envelope (E) proteins make up the majority of the viruses outer shell, while the Nucleocapsid (N) protein found inside the virus assists in viral replication. This small assortment of proteins, plus a few others, make up the bulk of the very simple viral structure (Weiss 2005, Li 2020). The novel coronavirus 2019 (COVID-19) shares a lot of homology or similarity with the original SARS virus that was discovered in 2002, genetically 80% similar, while being 76-95% similar for the major proteins listed above (Xu 2020). This allows researchers to draw a lot of conclusions from previous research on SARS-CoV-1, though we must be careful when doing so, as there are some known (and unknown) differences between the two viruses. The infection cycle starts with the virus gaining entry to the host, usually through mucus membranes of the eyes, nose and mouth. Once inside the virus often begins it’s infectious cycle by infecting vascular endothelial cells that line vessels throughout the body. While different viruses have different mechanisms by which they enter host cells, SARS-CoV-2 binds to the ACE2 receptor using its spike protein (same as SARS-CoV-1), allowing it to enter the host cell (Jia 2005, Walls 2020). Like most viruses, SARS-CoV-2 then goes through a multi-stage process by which it hijacks some of machinery inside our own cells to in order to replicate, escape and subsequently infect more cells in a continual cycle (Frieman 2008).
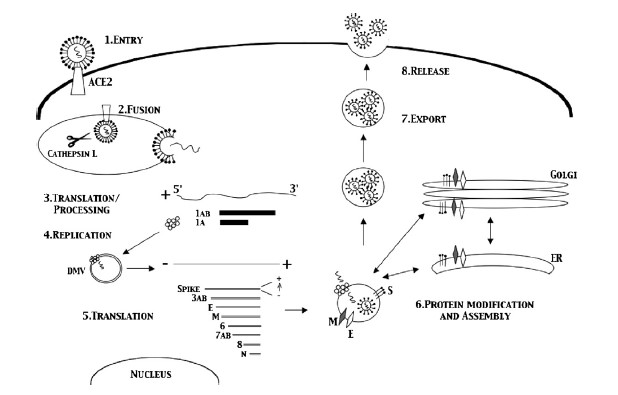
Early Cellular Response
Thankfully our body has a whole host of immune mechanisms it utilizes to deal with infectious agents of all types. Almost as soon as an invading pathogen has infected our cells the immune system starts going to work. The Innate Immune response is our constantly active sentinel, whose cells are constantly circulating all over our body just looking for foreign invaders to attack and kill. These innate cells use Pathogen Associated Molecular Patterns (PAMPs), or markers of foreign invaders, as the initial signals something is wrong and that it’s time to go to work (Li 2020). Some cells go to work directly attacking the virus and infected cells in an attempt to destroy the virus, others release signaling molecules known as cytokines and chemokines that recruit other cells to help in the fight (Frieman 2008), and some cells just go ahead and sacrifice themselves in an effort to prevent the virus from hijacking them, a process known as apoptosis (Lim 2016).
Symptoms: What and How They Manifest
While this system works well for many invading pathogens (why we are not sick all the time), allowing our body to control the infection, many viruses (and bacteria) have evolved mechanisms by which to evade, subvert and co-opt the immune response to their advantage. For SARS-CoV-2 it seems to be able to prevent the host immune system from activating one of it’s key anti-viral signaling pathways, the Type 1 Interferon pathway (Lim 2016, Li 2020, Frieman 2008). While it is not known exactly how the virus subverts this system a few hypotheses involve the Nucleocapsid protein (Lim 2016), other non-structural proteins (Lim 2016), and some of the SARS enzymes (Chen 2014). So by reducing the host immune response the virus is able to more effectively replicate and spread, leading to a more systemic infection. This is when we start to experience more of the hallmark symptoms of the infection; fever, sore throat, coughing, fatigue, pulmonary inflammation leading to shortness of breath and possible pneumonia and lymphopenia (a decrease in lymphocytes, more on that later) (Huang 2020, Zhu 2020). Most of these symptoms are a physical outcome of the body’s ongoing fight with the virus, trying to delicately balance destroying the invader, while preserving the host organs and system. The fever is the immune system’s attempt to raise the core temperature enough to burn out the infection. The sore throat/cough is an outcome of our immune system attacking infected cells of the airways and trying to expel the invader (mmm mucus), same for the pulmonary issues (initially, more on this later too). While many of these symptoms may be scary and uncomfortable they are often a normal part of our body’s healing process when dealing with a foreign invader. So under normal circumstances, it’s best to rest and let your body do it’s thing, unfortunately this doesn’t always go as planned, as we’ll find out in the following sections….
Adaptive Immune Response; Stage 2
In the previous two sections you’ve seen how our well intentioned Innate Immune system can sometimes fail leading to illness, thankfully the body has a backup, the Adaptive Immune response. This secondary wave of the immune response goes into action very soon after the initial infection (several hours to few days, infection dependent) and is mostly comprised of two cell types; T-cells and B-cells. When the levels of virus in the body start to rise, several of the innate immune cells can act as activators of the adaptive immune response, taking pieces of the virus to specialized activation centers know as lymphoid organs. These centers of immune activation are spread all over our body and are the primary site of pathogen specific antigen (virus pieces) presentation. The antigen presenting cells (Dendritic cells are most prominent) present the virus to the T-cells and B-cells as if locks in a door, allowing the T-cells and B-cells to go to work making specific keys (receptors and antibodies) that can attack and destroy the pathogen in a very focused manner. The outer proteins that make up the viral capid (proteins S, M, E) tend to be the most effective as this is what is visible to our body when intact virion are released (Liu 2017). So the body makes a whole army of these specific cells that traffic to the sites of infection; T-cells directly attack the virus and infected cells, while B-cells make antibodies that bind to parts of the virus, preventing them from entering new cells and marking them for destruction (Liu 2017).
These two arms of the Adaptive Immune response are also what comprise our immunological memory. Virus specific T-cells and antibody producing B-cells remain dormant in specialized lymphoid organs (sometimes they also remain in circulation), just waiting for the virus to turn up a second time. This time since they are already primed and ready to go, memory T-cells and B-cells start attacking the virus almost immediately, usually preventing the virus from spreading and preventing us from getting sick. Studies of SARS-CoV-1 have found both memory T-cells and memory B-cells (producing neutralizing antibodies) that are capable of rapidly responding to viral reinfection (Li 2020, Liu 2017, Channappanavar 2014). In human patients who recovered from SARS-CoV-1 infection anti-SARS antibodies and memory T-cells were found in most patients up to 24 months after infection (Liu 2006, Ka fai 2008, Liu 2017). While antibody responses did decline over time in SARS-CoV-1 patients (many undetectable at 6 years), memory T-cell responses were conserved for up to 11 years after infection (Tang 2011, Ng 2016, Liu 2017). Similar high quality neutralizing antibodies have been found in COVID-19 patients, but since the disease is so new the longevity of memory responses to this new virus aren’t exactly known. Encouragingly, since SARS-CoV-2 is so similar to the original SARS virus, and lab testing has even shown that their be might cross-reactive protection between the two diseases (Walls 2020), there is much hope that the long lasting memory responses seen for SARS-CoV-1 would also apply to those who have recovered from COVID-19. All of this evidence, both old and new, does inspire a lot of hope that a functional vaccine would both be likely and very effective in providing some duration of immunity from COVID-19, but how long remains to be seen.
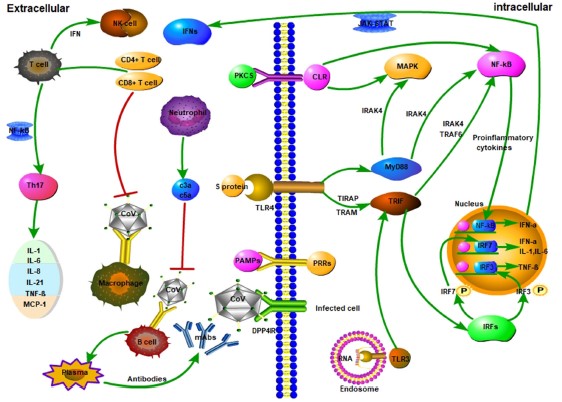
When the Immune System Fails, Severe Disease
The reason COVID-19 is such a scary disease, isn’t because our immune system has no problem fighting it off, but because in some percentage of the cases (uncertain, but estimates are as high as 10-20%) patients need to be hospitalized due to severe complications. If our immune system is so complex and so strong, why do patients with COVID-19 get so sick that they need hospital care? It comes down to numerous very subtle things this virus does that are different than coronaviruses that cause the common cold. One is the effect SARS-CoV-2 has on Type 1 Interferons mentioned earlier, reducing the body’s initial response to infection. Another early symptom seen in many severe cases is lymphopenia, or a loss of lymphocytes (notably T-cells) early on in disease (Huang 2020, Schmidt 2005, Weiss 2005). While the exact cause of this loss of T-cells is not known, it is hypothesized that the viral proteins may lead directly to T-cell death as a mechanism of immune evasion (Lim 2016, Li 2020). These mechanisms of avoiding immune detection along with the efficiency of viral replication can lead to an out of control infection very quickly.
But in the end, it’s only partially about the virus, and largely about an overexuberate immune response. In an attempt to catch-up to the wide-spread infection the immune response goes into overdrive, ramping up a lot of the inflammatory cells and signaling molecules that tell the body to attack the infection (Li 2020). This response does in fact kill the infected cells, but it also destroys lung tissue (primary target), vascular tissue, liver tissue and other infected tissues (Tian 2020, Schmidt 2005). This is often when the more obvious signs of pneumonia set in; the lungs fill with fluid, the efficiency of aveoli decreases (oxygen absorption) and breathing becomes very labored and difficult. This is the tricky thing about COVID-19, making our immune response more efficient would help prevent early infection, but later on would lead to increased tissue damage. But if we reduce the immune function of the body to prevent self-inflicted tissue destruction, we run the risk of allowing the virus to run rampant throughout our body. COVID-19 is a tricky disease to treat for these reasons, and because the disease severity has a wide range of outcomes for different people. In some, infection is very mild and asymptomatic, in others, their entire body shuts down as the virus (and immune system) destroys the host from the inside. The reason many comorbidities are important as risk factors for severe disease is that most of them either affect the immune system or lung function. Obesity, diabetes, auto-immune diseases all alter the immune system’s ability to function, making it harder to fight off the virus. COPD and asthma (though less prominent then thought) make the host pulmonary system more sensitive to damage caused by the virus and immune system.
But not all hope is lost! Because of the large body of evidence suggesting that SARS viruses create robust lasting immunity, this means a vaccine might be very effective at protecting most of the population. Also, now that there are many patients who have recovered from COVID-19, tests are underway to examine if using their plasma (containing antibodies) can help patients who are suffering from more severe cases of the disease (works for other viruses like Ebola). We also have several promising anti-viral agents that are already in clinical trials being tested against COVID-19, with hopes that one or more of them will help improve patient outcomes and be ready for use later this year. Unfortunately all of this does take time, meaning we won’t have a cure next month, but by slowing the spread of the virus, not only do we allow hospitals to manage the patient load, but we allow all the scientist out there to catch-up and produce much needed data, therapies and vaccines.
Thanks for reading. If you see any mistakes please bring them to my attention and I will correct them ASAP. If you have additional questions or want to discuss the immune response in more detail (this is a very high level overview) I’d be happy to do so via text or email. Stay safe and stay healthy.
Literature Citations:
Chan et al, Serological Responses in Patients with Severe Acute Respiratory Syndrome Coronavirus Infection and Cross Reactivity with Human Coronaviruses 229E, OC43, NL63. Nov 2005, Clinical and Diagnostic Laboratory Immunology.
Channappanavar et al, T cell-mediated immune response to respiratory coronaviruses. May 2014, Immunology Res.
Chen et al, SARS coronavirus papain-like protease inhibits the type 1 interferon signalling pathway through interaction with the STING-TRAF-3 TBK1 complex. Jan 2014, Protein Cell.
Frieman et al, SARS Coronavirus and innate immunity. 2008, Virus Research.
Huang et al, Clinical features of patients infected with 2019 novel coronavirus in Wuhan, China. Jan 2020, Lancet.
Jia et al, ACE2 Receptor Expression and Severe Acute Respiratory Syndrome Coronavirus Infection Depend on Different Human Airway Epithelia. Dec 2005, Journal of Virology.
Ka-fai Li et al. T cell responses to Whole SARS Coronavirus in Humans. Oct 2008, Journal Immunology.
Li et al. Coronavirus infections and immune responses. Jan 2020, Journal of Medical Virology.
Lim et al. Human Coronaviruses: A Review of Virus-Host Interactions. 2016, Diseases.
Lu et al. Immune responses against severe acute respiratory syndrome coronavirus induced by virus-like particles in mice. June 2007, Immunology.
Ng et al, Memory T cell responses targeting the SARS Coronavirus persist up to 11 years post-infection. March 2016, Vaccine.
Schmidt et al. Coronaviruses with a special emphasis on First Insights Concerning SARS. 2005, Birkhauser Advances in Infectious Diseases.
Tang et al, Lack of Peripheral Memory B cell responses in Recovered Patients with Severe Acute Respiratory Syndrome: A Six-year Follow-up Study. May 2011, Journal of Immunology.
Tian et al, Pulmonary Pathology of Early-Phase 2019 Novel Coronavirus (COVID-19) Pneumonia in Two Patients with Lung Cancer. Feb 2020, Journal of Thoracic Oncology.
Walls et al, Structure, Function and Antigenicity of SARS-CoV-2 Spike Glycoprotein. Apr 2020, Cell.
Weiss et al, Coronavirus Pathogensis and the Emerging Pathogen Severe Acute Respiratory Syndrome Coronavirus. Dec 2005, Microbiology and Molecular Biology Reviews.
Xu et al, Systematic Comparison of Two Animal-to-Human Transmitted Human Coronaviruses: SARS-CoV-2 and SARS-CoV. Feb 2020, Viruses.
Zhou et al, Clinical course and risk factors for mortality of adult inpatients with COVID-19 in Wuhan, China: a retrospective cohort study. March 2020, Lancet.